Written by: Jon G. Bell (belj@zhaw.ch)
Navigation menu
- Education
- Emissions & Air Quality
- Infrastructure & Services
- Meteorology & Climate
- News
- Physical Chemistry
- Projects
Volatile organic compounds (VOCs), such as benzene and formaldehyde, are characterised by high vapour pressures and, therefore, can easily adsorb to the surface of airborne soot particles, accumulating at the vapour-solid soot interface, within pores, or between the carbon core turbostratic graphene layers. These VOC-loaded, nanometric particles are extremely toxic, as they can easily be inhaled and settle in the human respiratory system, causing a range of life-threatening diseases.In general, soot particles from combustion sources are composed of a carbon core, an adsorbed hydrocarbon layer, and a soluble organic compound fraction, as shown in Figure 1A. However, soot characteristics can be highly variable, as their physicochemical properties strongly depend on the combustion conditions. Therefore, it remains a challenge to understand the mechanisms behind soot-VOCs interactions, as well as other atmospheric chemistry processes, in which soot is involved.
Typically, measuring sorption on aerosols requires expensive equipment and a high level of expertise, as it can be difficult to single out a specific adsorption process due to other interfering atmospheric processes (such as co-adsorption of water vapour). A more reliable and approachable alternative is the use of specialist vapour sorption systems, which allow measurement of interactions of vapours with soot particles on collected bulk samples. In the METENVIA group, we are setting up a dynamic vapour sorption (DVS) system (the IGAsorp system in Figure 1B), developed by Hiden Isochema (Warrington, UK). The DVS process involves measuring how much vapour (known as an isotherm) is adsorbed on soot particles and how quickly this occurs (sorption kinetics) at constant temperature. This will allow us to discern vapour interactions with soot particles, as a function of vapour chemical properties and soot porous structure, chemical composition, and surface chemistry. Ultimately, these studies are pivotal for the development of accurate emission chemical fate models.
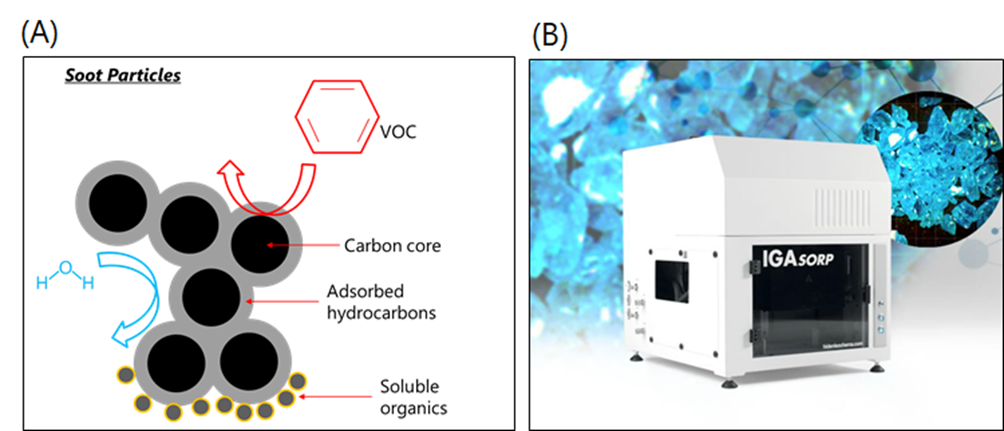
Understanding Dynamic Vapour Sorption: The Gravimetric Method. The gravimetric method is the operating principle behind the DVS system and is summarised by the schematic in Figure 2A, steps1-3. Initially, the partial pressure of a vapour (i.e. VOCs) is increased to the set point pressure and is then held constant (step 1). Subsequently (step 2), the solid sample (i.e. soot) adsorbs a quantity of the vapour and the weight changes to the new equilibrium position over time. The weight change is recorded and analysed, using a stretched exponential model, to predict the final equilibrium amount. The equilibrium points form an isotherm, while the weight relaxation between points results in kinetic data (step 3). The gravimetric procedure produces the simultaneous acquisition of sorption isotherms and kinetics, as shown in Figure 2 (B), respectively, at constant temperature, and for both adsorption and desorption processes. An example of experimental data from the IGAsorp is shown in Figure 3, with isotherms reported on the left and kinetics on the right.
The temperature dependence of isotherm data can be used to calculate isosteric heats of adsorption, using the Clausius-Clapeyron equation. This parameter is a fundamental measure of the strength of the electrostatic interaction (Van der waals), also known as physisorption, between the vapour (e.g. VOCs) adsorbate and the solid sample surface (e.g. soot). Similarly, the kinetic data can be fit to several mass transfer models (i.e. Fickian, linear driving force etc.) and the activation energy for diffusion can be calculated. These parameters can then be related to the soot composition, structure and surface chemistry, and consequently provide a deep insight into the sorption mechanism and the role of surface interactions on VOC partitioning.
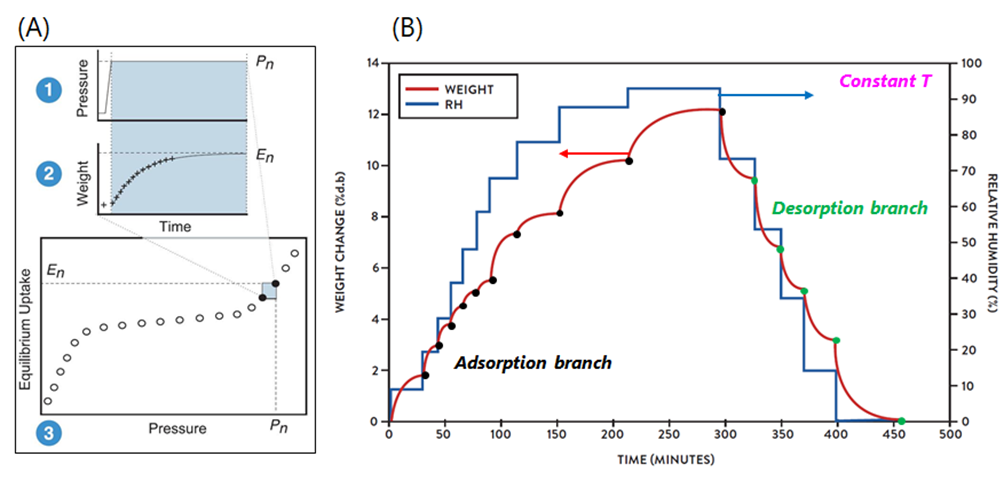
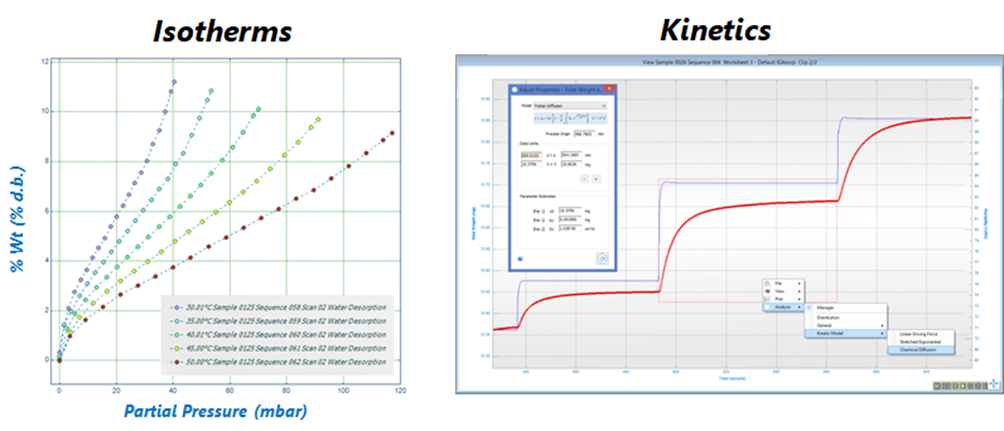
Figure 3: (Left) Measurement of adsorption isotherms between 30 – 50 °C. (Right) Experimental data showing the pressure set response and the resulting mass relaxation to equilibrium, resulting in both sorption isotherms and kinetics at constant temperature (used and modified with permission from Hiden Isochema Ltd).
Future Work. In the initial period, we will investigate the sorption characteristics of a range of combustion generated vapours, displaying different chemical properties, on soot particles with different morphologies and surface chemistries. There is a deficit of work that report kinetic data for VOC and H2O sorption on soot, which is a critical parameter for atmospheric and environmental fate modelling, as atmospheric systems are usually far from equilibrium, due to ever changing boundary conditions. The data derived from the DVS instrumentation will supplement additional characterisation data obtained from a wide range of analytical techniques. For example, soot surface chemistry will be studied with spectroscopic techniques, such as FTIR and XPS. It is our aim to combine these approaches in order to gain a profound understanding of the vapour-soot partitioning process, and to make further advancements in emission environmental fate modeling, atmospheric chemistry studies and even cloud nucleation processes.